From Earth orbit to the Moon and Mars, explore the world of human spaceflight with NASA each week on the official podcast of the Johnson Space Center in Houston, Texas. Listen to in-depth conversations with the astronauts, scientists and engineers who make it possible.
On episode 363, NASA experts discuss one of the most critical components of returning to Earth from space: the parachute system. Take a deep dive into how these parachutes were engineered and tested to bring NASA’s Orion spacecraft and crewmembers back home safely. This episode was recorded on Nov. 4, 2024.
Transcript
Host (Courtney Beasley): Houston, we have a podcast! Welcome to the official podcast of the NASA Johnson Space Center, Episode 363, “Orion Parachutes.” I’m Courtney Beasley, and I’ll be your host today. On this podcast, we bring in the experts, scientists, engineers, and astronauts, all to let you know what’s going on in the world of human spaceflight and more. Today we’re taking a closer look at one of the most critical components of returning to Earth from a space mission: the parachute system. And not just any parachutes. We’re talking about the advanced parachutes engineered to bring NASA’s Orion spacecraft safely back to Earth after returning from deep space. It’s hard to imagine enduring the extreme speeds and temperatures of a journey from lunar orbit as you reenter the atmosphere, relying on a parachute to guide the spacecraft through the home stretch of the mission. So what goes into creating such a reliable system and how do engineers test, retest, and prepare for the unexpected? In today’s episode, we’ll be speaking with experts Jared Daum and Enrique “Moe” Moeller, who have worked on this technology to get a behind the scenes look at the complex design, rigorous testing, and intricate mechanics that make it all possible. Let’s get started.
[Music]
Host: Okay. Well, Jared and Moe, thank you so much for coming on Houston We Have a Podcast today.
Moe Moeller: Thank you.
Jared Daum: Happy to be here.
Host: I wanted to start by getting to know a little bit more about both of you. Jared, let’s start with you. What is your title here at NASA and what is your role with Orion parachutes?
Jared Daum: So my title is Orion Parachute Subsystem Manager. So I guess technically, I’m in charge of all the aspects of the Orion Parachute System. The manufacturer, the integration, post-flight analysis. It’s been a long project. It’s been going on for well over a decade, maybe 15 years. I’ve only been a part of it for the last maybe 10 years or so. But yeah, everything to do with Orion parachutes. And then also I’m part of the larger parachute group where we also help our commercial partners be successful, SpaceX and Boeing, that use similar parachutes.
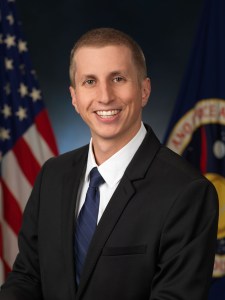
Host: Okay. So you’ve been on the Orion parachutes team for about 10 years, but you said you had worked on some different spacecraft parachutes before that.
Jared Daum: Yeah, well we do the Boeing, SpaceX, and Orion parachutes kind of in parallel right now. Prior to parachute work, I did a bunch of analysis on the GNC side, so some navigations analysis for the Artemis missions.
Host: Awesome. And Moe, same for you. What is your title here at NASA and what is your role with the Orion parachutes?
Moe Moeller: So I recently came into my current position, the deputy systems manager for the Landing and Recovery System. So that’s basically the overall management of the landing and recovery system, which covers the parachutes, but it also talks about the Crew Module Uprighting System, and that is the bags that upright the crew module after it splashes down, and it prevents it from tipping over and getting upside down in the waves.
Host: So interesting. Where are you both from and is NASA what brought you to Houston?
Jared Daum: Yeah, for me it is. So I’m from Illinois. Went to University of Illinois, actually started out as a music major. Switched over to aerospace engineering. And then I got a co-op down here, so co-op’s kind of like an extended internship where, you know, spend a semester down here, working semester back at school, back and forth. And then I got a full-time job here, starting in about 2011 and moved down here and been here since.
Host: What made you go from music to engineering?
Jared Daum: Good question. So I guess going into college, I kind of knew it was going to be one of the two. I’d invested a lot of time, you know, in high school practicing, participating in all the different kinds of musical aspects there. Just didn’t really want to give that up. So tried it out for a little bit, wasn’t really my thing. Eventually double majored, then switched over.
Host: That’s really cool. And Moe, what about you? Where are you from and what brought you to Houston?
Moe Moeller: I’m originally from Miami, Florida, and really, aside from the job NASA, it was really my wife. She’s the one that was from Houston, Texas. So after Miami, I joined the military and I was in for about 21 years. And we weren’t sure where we wanted to kind of, you know, start our next home. And next thing you know, this job came up in NASA and it also happened to be the same place my wife’s family was from. So, yeah, it was kind of a no brainer for us.
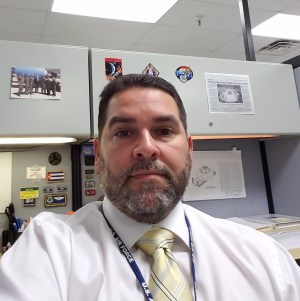
Host: How many years have you been here now?
Moe Moeller: Probably about 15 years.
Host: Awesome. Well, I hope the two of you are enjoying Houston outside of the humidity that we seem to constantly have.
Jared Daum: Absolutely.
Host: I was curious to know what other teams within NASA you both collaborate with to work on the parachutes.
Jared Daum: Yeah, really a lot of them. So, most people might not know a lot of the details about our parachutes. Maybe you think, you know, it’s like a big bedsheet up in the sky. But really, we have metallics, we have pyrotechnics. We interface with the vehicle structure. So really, we work with all those groups. The pyro folks, which I’m sure we’ll get into a little bit later. Structure, since we have lots of metallic parts, including the connection from the parachutes to the spacecraft, need to make sure that those are strong enough. All the way through safety, quality, quality folks are really big in our world since in parachutes we do a lot of touch labor, so we tie a lot of knots. We hook a lot of cords to other cords. And so that kind of touch labor has inherent variability, and that variability needs to be checked or bought off. So we have multiple layers of quality that come check all the work that we do to make sure that it’s as expected.
Host: Okay. I want to dive a little more into the details. Can you describe the parachutes for us from top to bottom?
Jared Daum: Sure. So each parachute type’s a little bit unique, but they all kind of have the same basic features. So starting at the top, we call it the vent. It’s the little hole at the top. So when you were a kid and you’ve built a parachute out of a grocery bag, maybe you cut a little hole in the top that adds stability to the chute. That’s a whole other interesting discussion to have. But anyway, we’ve got the vent at the top with a hoop that goes around it called the vent hoop that helps hold together the tension of all the radials. And so the radials are like slices of pie that go radially out from the vent from the vent down to the skirt of the parachute. And so each slice of the pie there we call a gore. And so for the mains we have 80 gores, so there’s 80 radials. And each gore is comprised of in the main’s rings and sails of nylon cloth. So rings are basically panels of nylon cloth that you can kind of consider flat. Those produce the drag that gets added up to put the force on the vehicle. The rings are at the top, followed by sails closer to the bottom. Now these sails are very similar to the rings except for they have a little extra length, which gives them some fullness when they’re inflated. So they look a little more conical if you will, or kind of like a wing, down towards the bottom. So the gores come down and meet at the bottom what we call the skirt. So there at the skirt is where we have our reefing lines, which are lines that control the inflation of the chutes along with our roofing line cutters. And that’s where the suspension lines connect to. So each of the 80 radials connects down to a single suspension line. And that suspension line is what you kind of see, looks like a cone coming off of the bottom of the canopy. And they come down to a confluence, which is the point that they all group together to form the riser, which appears to be like one big piece of Kevlar. But actually, it’s all 80 of those suspension lines bundled together. And those go all the way down to the vehicle around a complex metallic pin which connects that riser to the vehicle. So kind of in general, all those rings and sails of the cloth of the parachute generate the drag. That drag is transferred into the structural grid, which is those Kevlar radials and suspension lines, and those suspension lines and riser transfer that force down into the vehicle to slow us down.
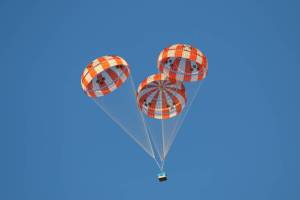
Host: Okay, that was really helpful for the big picture. And that’s a great segue into where I wanted to go next. I want to give our listeners a better idea of just how large one parachute is. Can you give us the size of a parachute and maybe a comparison to what it may be the size of that people would see in their everyday lives?
Moe Moeller: Sure. The Orion main parachutes, which are the largest parachutes are each 116 feet in diameter. And there’s three of them. So, you know, it’s about 10,500-square-feet each. But what really I think listeners want to know is how big they are. If you were to drape them down on the ground, all three of them, they would take up roughly the size of a football field.
Host: Oh, wow.
Jared Daum: Yeah. So most talking almost 11,000-square-feet, and you think like a standard house is maybe, you know, 2,000, 3,000-square-feet. So one of these main parachutes can, you know, cover up a few houses.
Host: And we use a football field for a size comparison for the International Space Station sometimes. So that’s really cool to hear that the parachutes are that large.
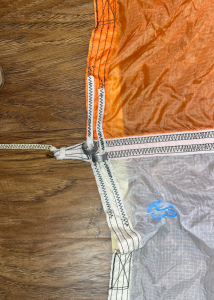
Jared Daum: Yep. And what really drives that is the mass of the vehicle that we’re trying to land. So basic aerodynamics and the aerodynamics of the parachutes pretty much state that, you know, the heavier the payload is the more force of gravity you have to counteract. And in order to do that for a given atmosphere, you just need more surface area or more drag area. And so bigger parachutes. So a smaller vehicle could have smaller parachutes. But for the requirements that we have for landing crew in the water safely, that’s the size of parachutes we need.
Host: For something that large, I’m interested to know what your workspace looks like. Is it a big warehouse or what do you guys work out of?
Jared Daum: Well, I hate to ruin it, but my workspace looks a lot like a desk with a computer.
Moe Moeller: Mine too. Mine’s just a cubicle.
Jared Daum: But that’s most of the time. So I think that’s really the beauty of our job is that especially like in the aerospace world, engineers like me don’t really have a lot of opportunities to do lots of cool analysis and decision making as well as like getting out in the field and actually doing stuff with our hands. So especially right now with Orion, since our flight rate is not very high right now, we do spend a lot of time at our desks solving problems, planning for the future. But kind of in the height of our development, like I would say Moe probably spent more than half the year out at our test range in Yuma.
Moe Moeller: Yeah, in Arizona.
Jared Daum: Yep. And, you know, I’m out in at KSC putting chutes on the spacecraft helping them get packed out in California. So it’s really a cool balance of being out in these factories or warehouses, like you mentioned, and also, you know, doing cool analysis work at our computers.
Host: Where do the parachutes live right now?
Moe Moeller: So the parachutes actually start off at Airborne Systems out in Southern California. Their facility is, for lack of a better word, huge, 140,000-square-feet. The tables that they have are probably the most impressive. When they start fabricating and I’m just going to use the mains cause the mains are the ones that are the most impressive ones just by their size. But they use a 50-foot by 50-foot table surrounded by seamstresses. And that’s how they sow the large pieces of nylon fabric into what we call the canopy. Once they get to that point, they typically, with a lot of different hands, will move the entire parachute itself to a riggers table or a packing table. And those tables are roughly about 200 feet in length. And that’s just a way of them just putting all the suspension lines, all of the canopy so forth, so that the riggers can actually put their hands on it and do everything from untangling the 80 suspension lines to removing twists in the lines. It’s just a lot of touch labor before it really gets into the hands of, you know, putting it into its deployment bag.
Host: That’s a great visual. I can really picture that all being created in my head. What kind of challenges would you say there are working with such a large item?
Moe Moeller: I would think it’s just moving it, getting it moved from one table to another, because at the end of the day, the contractor is a, it’s an assembly line. It’s an assembly line with a very large product. I mean, you start with something that’s, like we said, 116 feet in diameter, maybe about 160 feet long, and it gets, by use of a hydraulic ram, it gets packed into a small square block with probably the density of something close to wood. I mean, it is just a square block that weighs about 260 pounds at the end of the day.
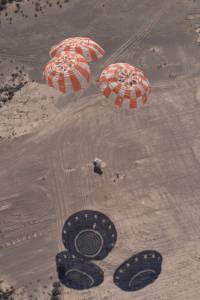
Jared Daum: Yeah. You can walk up to a packed parachute and knock on it.
Host: That’s so interesting.
Jared Daum: It’s pretty cool. So I guess I’ll add a couple things about kind of the complexities of working with something so big. Some people have described deploying these parachutes as an explosion of textiles, where we are basically building a 200-foot long, 10,000-square-foot structure instantaneously in the air during the mission. So really the planning and the testing that goes into that actual deployment is kind of one of the most amazing parts. Making sure that we design the parachute the right way and test it in a way that we have confidence that it’ll work every time cause we don’t know exactly how the spacecraft’s going to come back every time, can have failures, you can have different atmospheric conditions and really the chaos of all these textiles that behave differently than metallics. I mean, we can do a pretty good job of understanding metallics. You can torque a bolt, you can do stress analyses on metallics, a little bit more difficult with textiles. So everything that goes into the design and development of these chutes I think is really impressive. And then, like most said, getting out on the table, the rigors that actually do the packing of these chutes, they follow very precise procedures to make sure that everything is done the same way every time. And that’s really critical because there’s a lot to keep track of.
Moe mentioned, we don’t want to tangle the suspension lines. We have reefing I’m sure we’ll get into reefing in a little bit, reefing of the chutes. We have to make sure specific lines are routed in a somewhat complex way, but exactly right or else the parachute will fail. So just keeping track of all these little things where any one mistake could be catastrophic. These rigors are doing a really good job keeping track of everything, following the procedures. And like I mentioned, quality. We’ve got extra eyes looking all around, making sure that things are done the way we expect.
Host: That’s a great segue to my next question for you. It seems like any project we have at NASA, there are people all over the country and potentially world contributing. Do you know about how many people it are on the parachute team?
Moe Moeller: If I think back to, let’s say, when we started development early on, it started with obviously NASA, but the next level down was Jacobs Technology. Now it’s called Amentum. They recently had a merger. Jacobs had approximately, I think when we were our full strength, maybe almost 150 personnel. And that didn’t count, you know, folks from Airborne Systems that were the parachute experts. You’ve got folks at Roberts Research Laboratories, they’re the ones that develop and fabricate the cutters for the reefing lines that we use in the parachutes and there’s just so many vendors that we have to reach out to that just kind of build the team. But again, they’re just spread out throughout the country.
Jared Daum: Yeah. And you mentioned like geographically spread out, which is certainly true. Now we’ve got folks out at Kennedy Space Center that do our quality and that help do safety. We’ve got folks from the NESC at Langley. So really folks all over the country, but not only geographically, but like over time, so like generations. So we have experts from the Apollo days from the shuttle solid rocket booster chutes, Ares, X-38. So parachutes kind of are a black art, if you will. A lot of it’s experience space. There’s no parachute major, there’s no parachute classes in college. But what we do have are experts that have been doing this for decades and decades, and we have hundreds of years of cumulative experience on our team. And we really rely on those people that have done this successfully for so long to help steer us in the right direction, make sure that we’re thinking about the right things, telling us what has and hasn’t worked in the past. So we can use that to guide our decision making.
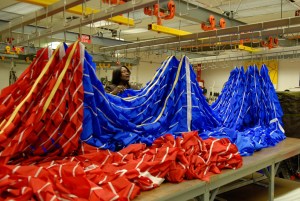
Host: With these future Artemis missions, we often hear Apollo being brought up. I was curious to know if your teams pulled on Apollo missions for the parachute design?
Jared Daum: Absolutely. Yeah. So like I mentioned, we have experts that work directly on the Apollo parachutes that guided us through the development. And a lot of what we do on Orion looks a lot like the Apollo parachutes because it turns out it’s the right thing to do. Similar with like the capsule design at the Orion capsule looks a lot like the Apollo capsule kind of at first glance, just because that’s the right type of shape for the aerodynamics of our atmosphere. So the architecture’s similar. We have a lot of more advanced materials just because of the development of things like Kevlar, Vectran over time. So we’ve also been able to take advantage of lots of new analysis tools. So we have a lot more computing power, a lot more ability to run complex simulations and run hundreds of thousands of Monte Carlo cases to try to figure out what we think these parachutes are going to do when we use them.
Host: You mentioned a few of the materials, but are the materials that are put into the parachute something you can go out and buy? Or are these special made materials just for parachutes?
Moe Moeller: No, these are pretty much, I guess what we would call COTS, commercial off-the-shelf. The nylon obviously is what the canopy’s made out of, and then let’s call it maybe the reinforce reinforced structures and the suspension lines are made of Kevlar. And again, those are just through just basic vendors. We do ask for a lot of, I would say, pedigree paperwork on it. So it’s not like just going to your local hardware store, picking up a roll and bringing it back. We asked for a lot of information, a lot of details on how that material is made, that strength, when it was manufacturer, you know, details like that.
Jared Daum: Yeah. And I guess we can get into a little more deeper on those specifics if you want. Like for example, the nylon that Moe mentions on the canopy or the broadcloth for our mains, not much different than the nylon that you make a tent out of or like an old school windbreaker. But like Moe mentioned, we really care about consistency and quality. So while the fabric and the manufacturing techniques may be similar to some nylon, you can just go to academy and buy a tent that’s made out of, we really care about it being done the same way every time, providing the evidence that it’s been done the same way every time.
Same with the Kevlar. So the structural grid that that Moe mentions made out of Kevlar primarily, cause that’s a high, we call it high tenacity. It’s very strong for its weight. It’s the same Kevlar that anybody can get from DuPont to make bulletproof vests out of or protective wear for race car drivers. But again, we really care about that quality. And not only the quality of the product that’s coming, but the selection of that product. So we don’t just go to DuPont and say, “Hey, throw us some Kevlar. Let’s build a parachute out of it.” We spend a lot of time optimizing the material choices to balance the strength to get the margins we need yet save mass and volume on spacecraft, cause we all know mass and volume’s the currency we work with, we want to make things small and light cause it’s hard to get stuff into space. But we need to have confidence that we’ve got the structural margins so the parachutes don’t catastrophically fail.
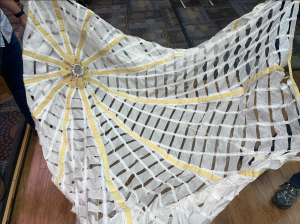
Host: And we talked a little bit earlier about the production of the parachutes. Would you say that it is kind of a combination of people working by hand and with machinery to put these together?
Jared Daum: Oh yeah, certainly. I would say primarily by hand. Sewing machines everywhere. So, Moe mentioned the Airborne Systems where they actually manufacture these, you can go out on the manufacturing floor and it’s just a constant whir of sewing machines, entire floor of them. A lot of the techniques to make these come from the garment industry, come from the sailing industry from long ago. So the way that we design our seams and our joints and the selection of these materials comes from experience in those industries. So, I don’t want to say primitive because that might put the wrong light on it, but I mean, it’s a bunch of people at a sewing machine sewing together a parachute.
Host: Yeah. I love the collaboration of all of the different strengths from everybody coming together to put these together. I wanted to know if there are any unique features in Orion’s parachute system that would distinguish it from those that we are flying now in low Earth orbit.
Moe Moeller: I would go back to maybe start with the Apollo era. You know, we talked about Apollo was kind of the foundation for Orion parachutes. I think our chief engineer Koki Machin, I think I remember having a conversation with him and he kind of did a comparison that Apollo, I think the crew module was about 13,000 pounds. So it had three 84-foot diameter parachutes, whereas Orion’s much heavier at 21,000 pounds. That’s why we’ve got the larger chutes. But when you start looking at the differences, when we first started designing the CPAS chutes, the risers were made out of steel. They weren’t Kevlar. And that was based off of, that was the technology at the time that Apollo worked off of. It wasn’t until a couple years into our designing that we realized that, you know, we should really take a good hard look at Kevlar. And it is just a reminder that how long ago Apollo was, Kevlar, I guess, wasn’t available to the very end of the Apollo era. So that’s why we just didn’t start off with Kevlar suspension lines. But again, that was a huge weight savings. I think the other item that makes the parachutes unique, I believe most parachutes use what’s called a reefing line steel loop, or a steel ring. So for some reason or other, and I believe it was driven by weight savings again, we went from steel rings to textile loops. And then of course that saves quite a bit of weight and mass on the parachutes themselves.
Jared Daum: Yeah, I think another reason we made that switch was we are finding that as we pack these parachutes to the density of wood, so we’re putting 140,000 pounds of force on the top of the packing plate. We are finding that these rings, which really looked exactly like a wedding ring, were kind of cutting through the nylon as they were kind of getting sandwiched around those rings. So we were starting to see damage in the parachute from those packing pressures. And then, I guess going back to your question of, you know, kind of low Earth orbit parachutes versus the Artemis mission coming back from the Moon or Mars kind of interestingly, the parachutes don’t exactly care about where we’re coming back from because by the time we get to the point of deploying the parachutes, we’re kind of at the steady state of the vehicle after the heat shield and the drag of the vehicle has dissipated all that energy. So really the vehicle and the heat shield kind of is the first stage of parachutes, if you will, to slow the vehicle down to our deployment conditions. And whether we’re coming back from low Earth orbit or coming back from the Moon, the parachutes be deployed about the same conditions.
Host: We’ve had a few missions to test these now, but I want to go back to Artemis I. What did your teams learn from the parachute performance on Artemis I?
Jared Daum: Yeah. We learned a lot. And also unfortunately, we may have missed out on some information. So one thing that’s super important for parachutes is learning from each mission. So this is a very good question. One of the best ways to do that is to recover the parachutes and inspect them to see what kind of damage there is. The parachutes will always tell some sort of story. So we spent a lot of time out at Yuma drop testing our parachutes. After every drop test, a thorough inspection of every square inch of that parachute marking down every nick, every burn, every little pulled fiber to try to understand what could possibly go wrong with these chutes.
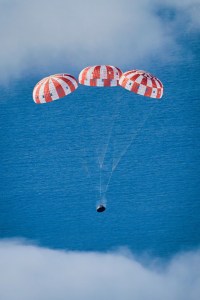
Unfortunately, for Artemis I, we weren’t able to recover any of the parachutes. They sank before the recovery team was able to get to them. So it’s kind of a missed opportunity there. But two of the things that we can use from Artemis I that are super valuable is, A. Imagery, and B. Data and data analysis. So on the data analysis side, we can use data from the vehicle, the IMU to reconstruct a trajectory and then try to infer what the parachutes did. And now we have load cells for each parachute. We have knowledge of what we expect the parachutes to do. So we can use all this to reconstruct the performance, try to understand how they behaved, and try to kind of play that forward into the future to see what we expect for future missions. The other really important thing is imagery. So all kinds of imagery to assess the parachute performance. So onboard imagery, that’s cameras on the spacecraft itself. We had some in the cabin looking out the windows to see like what an astronaut would see. We had some in the forward bay, kind of on top of the vehicle looking right up at the parachutes. Beautiful, beautiful imagery of the chutes being deployed and performing. And we can learn a lot from that. We can see entanglements; we can pull out timing from that to determine when the reefing line cutter’s fired. We can look at kind of the relative timing and area, if you will, of each parachute to kind of interpret how they’re sharing the load. So in addition to the onboard imagery, WB-57 and other air assets flying around with great camera equipment to get air to air imagery and also ground air or seed air in the case of Artemis video or cameras on the ships looking up and, and viewing the descent and splashdown. So we spent a lot of time looking through that video picking apart frame by frame to pull anything we can from it.
Host: Is that typical of a splashdown that you would potentially get there after the parachutes have already gone under?
Jared Daum: Yes. So let’s see. On EFT-1, we were able to recover two of the three mains, Artemis I, none. We’d like to do better. We’re working on doing better. Unfortunately, we don’t get a ton of practice cause our flight rate isn’t very high. I’ll give props to our SpaceX counterparts, who they also land in the ocean. They’re recovering every chute that they fly now. So they have a very good process, very good procedures, and they’ve got lots of experience getting those chutes back and we’re learning so much from them. So it’s something that we’d like to strive towards to be able to do the same. And we’re working towards that.
Host: And they have a more frequent cadence of splashdowns. So hopefully when NASA gets to that point, we’ll also be in the same boat. That’s great. Now that we’ve discussed the design in depth, I wanted to go over the testing. Can you walk us through the testing process and how you ensure that the parachutes are reliable under those conditions that they’ll face?

Moe Moeller: Gosh, we go back all the way. I know Jared talked about Yuma, Arizona, that’s where most, if not all of our full-scale testing took place. They started with small testing maybe out of a helicopter where they dropped a smaller parachute, like a pilot parachute or a FBCP parachute. And then all the way to the more complicated tests that we did during the end of development and qualification testing where we were dropping vehicles weighing close to the 21,000 pounds of the Orion capsule. And we did a lot of testing out of military cargo planes. And I would say probably the most challenging part of it was just the sheer size of the capsule itself, or the mock-up capsule. We called it a parachute test vehicle at the time. And getting to those altitudes that, you know, typically we don’t do airdrop testing from. But we managed to get up to 35,000 feet, which was optimal at the time for that side sort of testing. And I would say we did a total of, I think, almost 18 developmental testing and about eight qualification testing. So it took about eight years to get to the point where they were completely certified for human spaceflight.
Jared Daum: Yeah. And so breaking that down a little bit, the development testing that Moe mentions is us kind of shaking out the design, kind of seeing what works, making design change decisions as we go through it to really optimize and guarantee the consistent performance that we want. And then getting into the qual test campaign, the final eight tests, that’s kind of like our final exam where we’re not changing anything, we’re just demonstrating that these parachutes work in a whole set of conditions, whether that’s high speed, low speed, failed chutes across the board. That’s really our demonstration that the system is ready to go.
So we’ve got 11 parachutes on Orion. They’re pretty complicated, lots of detail that we haven’t gotten into yet. But to give Moe some credit, a lot of what he did out at Yuma was to get the test vehicle to the test point to start that test. And that in itself was extremely difficult. So Orion coming back from the Moon or from low Earth orbit, it decelerates with the heat shield, it gets to about 35,000 feet or 30,000 feet. And we start the sequence. We need to make sure that we start that sequence at approximately the same conditions in our drop test. And so 11 parachutes on the actual flight vehicle, but we had at least that many parachutes that we needed to get us to that test condition. Whether that’s to pull the vehicle out of the airplane, to recover some of the test equipment that that was used to pull it out, wind packs to measure the atmosphere and the wind and the column of air that we descend through. Really a lot of analysis, a lot of hands-on rigging work to really make that happen. And I think that’s where a lot of the experience and success of our team really shined was out there Yuma getting all that rigged up.
Moe Moeller: And getting back to your point about imagery being so important. I mean, just for the testing that we did in Arizona, I mean, aside from the KTMs, these very, very high-powered video cameras that were on the ground, we called what we called the flying circus. I mean, you had the military cargo plane that had the test vehicle in it, but you had a chase plane that could be a small Cessna to, I think a couple times we had an F-15 from Edwards as our chase plane multiple other aircraft taking video and imagery, and then helicopters from Yuma proving ground taking imagery as well.
Jared Daum: So at a couple different altitudes, too, coordinating all that.
Moe Moeller: A couple altitudes. Oh yeah, it was, we actually had our own air boss like the Navy uses. So yeah, it was just an incredible array of just imagery, video all happening within a matter of four minutes.
Jared Daum: Yeah. And so that’s like the high-level view of all these things happening. And then the complexities like going down into the avionics. So like our avionics team that spent all that time out there, Yuma, making sure our flight software, had all the timings correct for all the sequencing, making sure our cameras were working just, yeah. Insane amount of work.
Moe Moeller: I think at one point, I think I counted like 22 different high speed or high-definition cameras on our test vehicle. And we were pretty happy when typically, they would all work. Every once in a while, you had one that didn’t work, just because probably didn’t like being shoved out from 35,000 feet out of an airplane. But for the most part, that’s why we had so many cameras for the redundancy.
Jared Daum: Just like parachutes redundant.
Moe Moeller: Just like parachutes.
Host: That’s really neat. And kind of like you were saying about the Artemis I mission, that imagery and video is probably so crucial to your testing process.
Jared Daum: Absolutely critical. Yep. Yeah, so critical that we had requirements for it, and we had to, in the end, before we could certify the system, show that we have evidence that, for example, we called it orderly deployment. So when we mortar out a drogue parachute or a pilot parachute, we want to make sure that it gets to its inflation conditions every time. And the best way to fail a parachute is to put it through a disorderly deployment or you kind of, in other words, you have parts of the parachute coming out at the wrong time and things get tangled. So we really relied on the video from drop test to verify the orderly deployment of all of our parachutes.
Host: Can one of you or both of you describe the kind of forces that the parachutes are made to withstand?
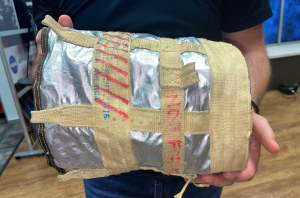
Jared Daum: Yeah. Oh, this is a good question. I like this. Okay. So a main parachute is designed to withstand about 46,000 pounds. That’s the highest loads that we expect. We predict that load using our complex simulations that use empirical models that we’ve derived from all of our data, from our testing, along with Monte Carlo analysis approaches to guess at or to predict these peak loads. But that’s just a load that an entire canopy produces it. And what we really care about is what is going to break in a parachute.
So we’ve got one big parachute, but it’s comprised of lots of smaller elements. So for example, the suspension line. So you’ve got the canopy of the parachute, it looks kind of like a hemisphere. And then coming down from that, in the case of our mains, you have 80 suspension lines that go around the circumference. That’s what’s going to break. So we need to figure out how do we assess the margins in each one of those suspension lines? Well, the first and easy way to do it, would be, well, you take your parachute load, let’s call it 46,000 pounds divided by 80, and that’s the load in each of those lines. In the perfect world, maybe, yes, but we live in the real world where there’s physics and chaos. And so the load’s going to be unequally distributed along those lines. We call it asymmetrically distributed. So we have complex models, again, based on test data that we’ve gathered through a lot of drop testing to try to predict based on the canopy load, what the load is in each one of those elements. And so we have to do that throughout the entire canopy. Every element that we have, we need to know, A. How strong it is, which we do through testing and analysis. And then B. What is the load that’s going into it? We do through analysis and models to determine what our margin is. So, yeah, the short answer is 46,000 pounds on a main, but it gets really complicated when we try to break it down to determine how safe and how much margin we have in these parachutes.
Host: Do you know about how fast Orion will be traveling at the time the parachutes are deployed, and how much the parachutes slow it down?
Moe Moeller: In the end, the parachutes slow the vehicle down to about 30, I want to say 30 meters per second, or 30 feet per second, 30 feet per second. I think nominally though, it gets down to about 20 feet per second.
Jared Daum: Yeah, I think on the order of like 17 miles per hour is our touchdown. So, the first chutes, I guess this would be a great time to talk about the sequence of parachutes. So we’ve talked about, there’s 11 parachutes. We haven’t talked about what they’re like. So let’s get into that. So we have four different types of parachutes. The first set of parachutes are called the forward bay cover parachutes, and their job is to lift the cover off of the vehicle. So the forward bay cover parachutes, they are all Kevlar, they’re seven feet in diameter and we mortar deploy them out of the cover. So literally there’s pyrotechnic cannons in the cover that shoot these things out at a hundred feet per second out into the airstream. The three chutes inflate and help lift the cover off the vehicle. That happens at about 350 miles per hour. So the vehicle has slowed down from orbital or lunar velocities of, you know, like 20,000 miles an hour down to about 350, just based on the drag from the heat shield. Those forward bay cover parachutes lift off the forward bay cover to expose the rest of the parachutes. Right after that cover leaves two drogue parachutes are mortar deployed out again, big cannons, I’m talking, you know, a foot and a half in diameter. They shoot out at about a hundred feet per second. These drohue parachutes are nylon and Kevlar. They’re about 23 feet in diameter, and their job is to slow the vehicle and stabilize it down to about 150 miles per hour. So we ride those drogues down to about 6,000 feet, at 6,000 feet more pyrotechnics.
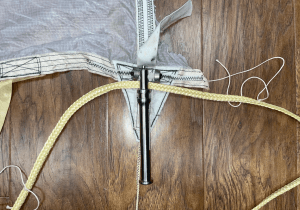
We have these riser cutters that are big blades that are propelled kind of like a big bullet, and they cut these Kevlar lines to let the drogues fly off. Once the drogues are gone, three pilot parachutes are deployed, again, mortar deployed. Those three pilots are about 11 feet in diameter. Again, nylon and Kevlar. Their only job is to lift up our big main parachutes. The big mains are too big to be mortar deployed. They’re about 280 pounds each. We’ve got three of them. So those pilot parachutes, their job is to release the retention of those main parachutes and then lift the chutes up and deploy them. And those main parachutes trail behind the vehicle about 200 feet. So the pilot parachutes pull them out riser first, followed by suspension lines, followed by the canopy for about 200 feet. And then they can open up and start to inflate. So that happens around 150 miles per hour. And then that they slow us down gradually to about less than 20 miles per hour when we have three good main a little faster if we have a failed main.
Host: I wanted to know about how the sequence is started. Is it an automatic part of the spacecraft or does someone have to press a button?
Jared Daum: Yeah, this is a good question. I like this. It’s automatic. It is automatic. It’s done by the fly software. The crew always has the ability to override things that the flight software’s is doing. So that said, the forward bay cover parachutes are done based on altitude. So we deploy those chutes at a certain altitude. I think actually it’s 23,000 feet. But we do know that the shape of Orion is unstable once we get to subsonic speeds. So eventually as Orion slows down, it wants to tumble. So we want to get these parachutes out before Orion starts tumbling. So we have a goal altitude that we try to get to before we deploy the FCPs, but our flight software is monitoring the vehicle to make sure that we don’t start tumbling. So we call it a smart deploy or a bailout logic. We’ll ride the heat shield down to 23,000 feet unless the vehicle determines that it’s about to tumble, that’s when we’ll bail out early, start the parachute sequence so that we don’t start flipping. So the forward bay cover parachutes are deployed, the drogues come right after that, immediately after that, and then we ride the drogues down to about 6,000 feet. Ideally, we can get pretty low there. The lower we ride the drogues, the less time we have on the mains. The less time we have on the mains means the closer we can land to our intended point. Because once under the mains, we’re descending pretty slowly. The winds are able to push us wherever they want. So the lower we can deploy the main safely the better. But we’ll ride those drogues down. And this time, instead of a bailout logic, we are really looking for an optimal point to cut away the drogues and deploy the mains.
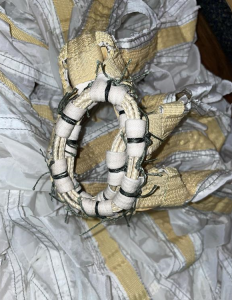
So since our pilots have to pull the mains 250 feet out before they can start to be deployed, that’s a couple of seconds there. Plus, maybe a second that it takes to deploy the pilots. That’s about three seconds or so that the vehicle doesn’t really have any parachutes attached to it. So it’s at risk of tumbling, if it were released kind of as swinging like a swing set. You want to release the drogues when you’re kind of at the apex of that swing, even though you’re not pointed, you know, straight down. Ideally you have low rates. And those rates are what we care about cause if we were to cut away the drogues at the bottom of the swing, the vehicle will want to tumble for those three seconds and deploying parachutes with an upside down vehicle. Not good. So our flight software is watching the vehicle rates and calculating at optimal time to release those drogues. While we’re kind of at the top of that swing, we’re at the lowest amount of vehicle rates, so that for those three seconds that we’re deploying the mains, we have a pretty stable vehicle as falling.
Host: I assume the crew inside can feel a difference immediately when the parachutes are deployed, right?
Jared Daum: Oh, yeah. And they can hear them, too. So, we’ve heard stories from astronauts that say, you know, one of the most reassuring things that they hear in the whole mission is the boom boom of the drogue mortars deploying those shoots.
Host: I’m curious to know if there’s a reason for the orange and white stripes that are used. Does that go back to a tradition or is there a specific reason for that?
Moe Moeller: I think it a lot has to do with visibility. You want to be able to see those parachutes from whatever distance the ship is at. And I would say, I think the orange is International Orange. So it’s a very common color, I think for recovery operations out at sea.
Host: I know everyone always has a big sigh of relief. The second that the parachutes are deployed in the orange and white is just such a staple that everybody remembers.
Moe Moeller: Absolutely.
Jared Daum: Yeah. And some more interesting stuff about the colors. So you can see the orange and white, but if you look closely, each of the three parachutes is a little bit unique. So they’re kind of, we call them candy striped. They kind of look like pieces of a pie. There’s actually clockings on the chutes so that we can visually look and see the difference between the chutes and look at them and know which one is what serial number and what part number. So if you look closely, you can see one chute’s got the pie pieces that are, you know, straight colors. One chute is kind of clocked such that orange turns to white. And then the third chute has two clocking points where orange turns to white turns to back to orange.
Host: Super cool. How does the parachute system ensure redundancy? Are there any safety mechanisms in place to handle any sort of malfunction that could occur?
Jared Daum: The redundancy is built in, so there is nothing that we can do reactively to kind of help the parachutes. But proactively, we have designed redundancy in per our requirements. So, I went through the four different stages, or four different types of parachutes that we have. We are designed that we can have a failure in each of those four types. So we can lose one FBCP and lose one drogue and lose one pilot and lose one main and still safely land the crew. So there’s redundancy kind of at the higher level there, but then we can get into the actual design of each individual parachute. So something we didn’t touch on yet is the reefing of the parachutes. So not only do we use pyrotechnics to deploy the parachutes out into the airstream, but there’s actually pyrotechnics embedded in the parachute that actuate after they’ve been deployed. So, again, taking the mains for example, cause it’s kind of the most visible example of this. When we initially deploy the mains, you can watch some video and for the first eight seconds they kind of look like maybe a cigar shape or like a really tall light bulb. That’s because we have a line at the skirt of the parachute that’s constricting the diameter of it. And we do that to control the loads and to kind of organize the inflation and make sure that we have a healthy chute. If we had gone straight to full open, that area is so big and we’re moving so fast that 150 miles per hour that would pretty easily destroy one of our chutes. So we’ve got this line constricting the diameter of the opening of the parachute. It’s got this long shape. And that pyrotechnic reefing line cutter in there. It’s a pyrotechnic fuse followed by a pyrotechnic cutter, kind of like I described earlier, the riser cutters that sever the drogue riser lines. These reefing line cutters sever that reefing line that’s constricting the skirt. So the first stage is eight seconds long. So we have an eight second fuse that burns. It cuts that line. There’s redundancy there. We have two redundant cutters for each line that we have. So, if one cutter fails to fully actuate, we’ve got that redundant cutter there. So after we cut through that first line, that skirt pops open just a little bit and we change shape of the parachute. And so for the next eight seconds, it looks more like a kind of a fat light bulb shape. And we ride that until we get to steady state. It takes about eight seconds. And then another set of cutters that now have been running their fuse for 16 seconds, those sever that line. And then that’s when we can eventually get to that full open. So there’s redundancy even within the parachutes there with those reefing line cutters. We also consider some of our elements in the parachute safety critical, where if we fail a single one of those elements, the parachute fails. So for example, the vent hoop that goes up around the apex of the parachute, it’s basically like a belt, a very strong belt that keeps the top of the parachute together. If we fail that vent hoop, we consider the parachute failed. We think it’s just going to rip open. So we design extra margin into that vent hoop. But other parts of the parachute are kind of redundant in that we believe that if some parts of the parachute fail, we’ll still have enough drag area for that parachute to performance function.
Host: When you ask most people what they’re most looking forward to about the Artemis II mission, they say splashdown for good reason, successful mission. But after Orion splashes down, how are the parachutes recovered? We went into it a little bit, but I just wanted to see are there some people on the recovery team who are parachute specific?

Moe Moeller: I would say you’ve got the U.S. Navy that provides support for the recovery. They’ve got smaller boats that will actually go out to the capsule itself. Obviously the first priority is always the astronauts, making sure that they’re safe, making sure that they’re actually taken out of the capsule as soon as possible. But we’re hoping to have a second team try to recover the main parachutes. There’s a lot of safety features that they have to go through before they could start that recovery. So a lot of times it’s spent time away from the crew module. But we’re trying to find different ways to see about expediting that time cause if, like Jared mentioned, we weren’t able to recover any of the parachutes or any of the mains for Artemis I and that’s just because they just sink very quickly. Once the capsule splashes down, after a few seconds, the risers for the mains are cut away using those riser cutter assemblies. So there’s nothing anchoring them to the crew module anymore.
We’re hoping to use maybe small buoys to maybe get the crew out to like let’s say a main, put the buoy on and then stand back in case there’s any hazardous liquids or hazardous smoke or anything like that, that they’d have to do some sort of prolonged keep out for the recovery. But other than that, I think the idea is always to try to recover the three mains. The other parachutes, I think because just where they land, they land so far away that by the time the crews get to it, they’re well under the water.
Jared Daum: Yeah. And so kind of on the safety aspect, not only do we need to consider any hazardous substances at the recovery of the vehicle, but we also need to consider all the stuff that’s coming down from the sky. So I mentioned the parachutes start deploying above 20,000 feet, and they create a lot of debris. There’s the lids to the mortars, there’s the bags that the parachutes were packed in all kinds of stuff coming off the vehicles starting, you know, above 20,000 feet. So we’ve got crews on the ships, on the sea. We’ve got folks in aircraft and hilos. And so we have a pretty sophisticated simulation, if you will, to predict where all of these individual elements of debris will be so that we can plan accordingly and have the different assets that are staged at different altitudes, be able to move in at the right time such that they can get as close as they can, but do so safely after the debris has fallen. And same with the crew and the boats. We don’t want them to head on towards the vehicle. And then, you know, something fall on top. So lots of planning goes in there, too.
Host: For the three mains, if you were able to get there in time before they did go under, would that be reusable?
Moe Moeller: No. Well, technically they could be reused, but I believe because there’s so much that goes into the production of these parachutes, our plan is not to reuse any of the parachutes. We’ll go through, give them the full inspection, probably keep them around for quite some time in case we find something later in the video that we didn’t see. But for the most part right now, the plan is always to have new parachutes, new cutters, new everything for each Artemis mission.
Jared Daum: Yep, that’s right. I will say in general though, parachutes are reusable. So like sports skydivers reuse their rigs all the time. The military reuses parachutes, some of our commercial partners reuse parachutes under some certain circumstances. So once a parachute falls in the ocean, I mean, it’s still a parachute. I kind of like to use a baseball anal analogy here. So like in the little leagues, you know, a pitcher throws a ball, it hits the dirt, a kid hits the ball, it gets scuffed up, it gets deformed, but it’s still a baseball, right? And little leaguers can reuse it. But in the big leagues, anytime a ball hits the dirt, or a batter fouls it off new ball, just because these pitchers are operating under such slim margins, they’re throwing it so hard, so close to these batters that any minor differences in the ball could really affect the outcome. That’s kind of like what we’re dealing with our parachutes. A sports skydiver or a lot of military applications, they can afford to design in higher margins in their parachute’s stronger materials. But we are operating under such strict constraints on mass and volume and trying to get, you know, every pound out of these 46,000 pound parachutes that we can’t really afford to incur degradations that we’re not expecting. So things like salt water or abrasion from the operation of the chutes down to fatigue degradation from repacking, the chutes over and over, those are things we have to consider that might eat into our razor thin margins that we’re operating under.
Host: How many parachutes do your teams have ready to go at any given time? Is it just the amount needed or do you make extra?
Jared Daum: So they’re very expensive and very difficult to build, so we plan very carefully. Right now, we’re in the process of manufacturing chutes through Artemis VIII. So that’s pretty cool. Artemis II parachutes are installed on the vehicle ready to go. Artemis III parachutes are packed and ready to install, and so we’ll head out there. I think the plan now is in January we’re going to go put the Artemis III chutes on the vehicle. We’ve got Artemis IV and V packed and ready to go. Just waiting for the word to go put them on a spaceship. And then, like I mentioned, we’re manufacturing and packing VI through VIII.
Host: How far before a lunar mission do you typically pack the parachutes on the spacecraft?
Jared Daum: Packed, quite a while. So the parachutes themselves really don’t have a shelf life. I think we certify them for maybe 15 years or so. There’s lots of experience like through the Navy with ejection seat parachutes that, you know, they really can last a long time. The limiting item in these chutes is the reefing line cutters. So those pyrotechnics that we store in the parachute that operate after we deploy the parachutes, those have a limited shelf life. Just cause the pyrotechnics degrade over time and their performance will change. And we really need to have predictable, consistent performance out of those. So those really are kind of our limiting life item. Anywhere, let’s call it seven years, is kind of the limit for these pyrotechnic devices. So we are comfortable having packed chutes for about seven years before they fly. As we get beyond that, then that’s when it’s going to take a little more effort, analysis and discussion to determine the risks and kind of balance that with unpacking and repacking.
Host: Okay. Artemis II coming up. I want to hear from both of you. What are you both most looking forward to on the parachute side?
Moe Moeller: Oh, splashdown. I mean, not to take anything away from, you know, the mission, it’s just seeing those parachutes completely inflate. I mean, whether or not we’ll get to see the drogues or the pilots, that’s a bonus. But the mains, just seeing the mains completely open, the nice orange and white colors, that’s of my favorite part of the whole mission.
Jared Daum: Yeah, that kind of brings me back to Artemis I. I was fortunate to be in the MER, kind of the engineering version of mission control during this. And so I was responsible for parachutes and helping the recovery folks. And it’s kind of like as the vehicle’s reentering kind of goes through blackout as it’s coming out of blackout, all eyes are on you and everyone’s holding their breath waiting for those first parachutes. And kind of like I mentioned, you know, the astronauts, they always say, you know, the most comforting sound is the sound of those drogues. Everyone’s just, I’m watching the data, I’m watching the video, just waiting for those first chutes to come out. And once they come out, then the adrenaline starts going and you go through the sequence and I’m picking out every little thing I can. Yeah, just that rush of the complex sequence that happens over the period of a couple minutes culminating in that splashdown. That’s really cool.
Host: So exciting. Well, I learned so much today. I’m also looking forward to splash down because now there’s a million things I’m going to be looking for in the parachutes that we discussed today. So exciting. Anything else you’d like for our listeners to know about the parachutes before we close that we didn’t touch on?
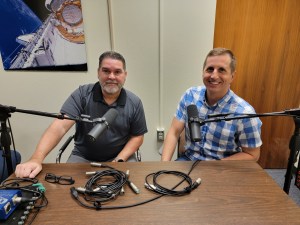
Moe Moeller: I think for me, when you look back at the amount of time it took Orion to develop and qualify the chutes, it was about between 12 and 15 years. And that might seem like a long time for parachute development, but I think the thing that makes NASA unique is the fact that we were able to take that data, lessons learned, all of the stuff that we learned from that, and provide that to commercial crew partners. And I mean, at the end of the day for commercial crew, the bottom line is the bottom-line cost. And being able to share all of the, that knowledge and all that data with them, I think it really helped them speed up their process and getting their parachute certified for human spaceflight.
Jared Daum: Yeah, and maybe I’ll just touch on the team that it took to get to where we are. I kind of feel, especially with parachutes, just the amount of unknown unknowns that we have to work through and be prepared for really makes the teamwork aspect of this so critical. Everyone’s ideas are important. We really have to consider all of the potential outcomes and really rely on each other to cover our specific areas and our specific tasks. So the trust that our team has had and the fact that we’re spread out so large, so much touch labor that goes into this and that it’s all worked out as well as it has been, is really a testament to the people that got us started and pushed us through to the end here.
Host: Teamwork makes the dream work.
Jared Daum: Teamwork makes the dream work, and we’re about to see the dream work on Artemis II.
Moe Moeller: I can’t wait.
Host: We are. Well, Jared and Moe, thank you so much for joining us today. This was such a fun one.
Jared Daum: Thank you.
Moe Moeller: Thank you.
[Music]
Host: Thanks for sticking around. I hope you learned something new today. The Orion Parachutes interview took place on Nov. 4, 2024. You can find out more about Orion parachutes and future Artemis missions on nasa.gov/Artemis. And Jared brought along some test articles for this episode that you can check out at nasa.gov/podcasts. You can also check out nasa.gov for the latest news and find more episodes at nasa.gov/podcasts. You can follow Johnson Space Center on Facebook, X, and Instagram. Use #AskNASA on your favorite platform to submit your idea and make sure to mention it’s for Houston We Have a Podcast.
This episode was recorded Nov. 4, 2024. Thanks to Will Flato, Daniel Tohill, Dane Turner, Abby Graf, Jaden Jennings, Dominique Crespo, and Laura Rochon. And of course, thanks again to Jared Daum and Moe Moeller for taking the time to come on the show. Give us a rating and feedback on whichever platform you’re listening to us on and tell us what you think of our podcast. We’ll be back next week.
This is an Official NASA Podcast.