Laboratory of Countermeasures Development
The Laboratory of Countermeasures Development, under the direction of Dr. Yuri Griko, is addressing key challenges associated with use of pharmaceutical medications in spaceflight missions; gaps in efficacy, stability, and side effects; issues related to the potential polypharmacy problems and the assessment of long-term spaceflight on drug metabolism and clearance due to a variety of physiological changes in the human body that are caused by exposure to microgravity.
The laboratory’s current research includes: (1) rational development of radioprotectants for collagenized tissue damage; (2) development of a novel class of radioprotective immunomodulators with high efficacy; (3) evaluation stability, integrity, and therapeutic effectiveness of drugs used in NASA’s human spaceflight program; (4) evaluation of new medications available on the market with the potential to work in a spaceflight environment, including weightlessness and space radiation, and in alignment with the data provided by FDA; (5) development of novel metabolic control technologies to enable reversible induction of a state of suspended animation in non-hibernating animals and potentially in humans.
Current Projects
DETERMINATION OF THE IN-FLIGHT PHARMACEUTICAL STABILITY OF LIDOCANE
The stability of medications carried aboard spacecraft during long-term space missions, such as a mission to Mars, is of paramount importance to enable the treatment of medical illnesses that may affect astronauts in space. Medications degrade at a faster rate aboard the International Space Station than on Earth, typically 1-2 years. The exact cause of accelerated degradation during spaceflight is unknown, although radiation exposure is suspected to be the main contributing factor.
In our laboratory, we use a combination of Differential Scanning Calorimetry (DSC) and Ultraviolet (UV)-spectrometry to determine the enthalpy of lidocaine–a local anesthetic and antiarrhythmic drug that is contained in space station medical kits. Changes in enthalpy can indicate drug degradation. The results from this study will be used to develop strategies for increasing the pharmaceutical stability of lidocaine.
IN-FLIGHT PERSONALIZED MEDICATION MANAGEMENT
Project in collaboration with DiaCarta Inc., Richmond, CA (Dr. L. Peletskaya)
Currently, the selection of medications for treating astronauts during spaceflight missions is primarily dictated by the need to efficiently treat the widest possible range of physiological conditions and illnesses with a limited set of medications. Dosage and recommendations on the combination of drugs are based on the assumption of genetically-equal drug sensitivity and unchanged metabolism. To our knowledge, there has been no pre-flight drug sensitivity testing on a genetic level for any of the previous manned NASA space missions.
Although many of the common, binary drug-drug interactions are, most likely, already considered in the International Space Station Medical kit composition, multi-drug and multi-drug-gene factors are not incorporated in the medication selection or prescription. Furthermore, due to the physiological changes occurring in microgravity environments, astronauts might be susceptible to potential increased drug toxicity as a result of decreased clearance of numerous drugs.
In particular, perturbation of CYP450 enzymes which contribute to the hepatic metabolism of the majority of drugs may have significant effects on therapeutic efficacy and increase treatment-related toxicity. The genes encoding the CYP450 enzymes are highly variable in humans. Inheritable variations of CYP450 hepatic metabolizer enzymes and transport proteins play a crucial role in the inter-individual variability of drug efficiency and risks of adverse drug reactions. Additionally, there are some reports that document changes in the levels of production of drug-metabolizing enzymes in microgravity. These data can be extrapolated to provide reasonable assumptions of decreased levels of expression for most CYP450 enzymes in the human body during prolonged space travel.
If the prescribed medication regimen is not fully effective or causes undesirable side effects, the ability of the astronauts to function and maintain peak performance levels during spaceflight could be seriously compromised. Therefore, technologies capable of predicting and managing medication side effects, interactions, and toxicity of drugs during spaceflight are needed.
We are working to develop and customize for spaceflight applications a Personalized Prescribing System that would provide a comprehensive, non-invasive solution for safer, targeted medication management for every astronaut crew member resulting in safer and more effective treatment and, consequently, better performance. The Personalized Prescribing System will function as both decision support and record-keeping tools for flight surgeons and astronauts in applying the recommended medications for situations arising during flight missions. The information on individual drug sensitivity will translate into personalized risk assessments for adverse drug reactions and treatment failures for each drug from the medication kit as well as predefined outcome of any combination of them. Dosage recommendations will also be made individually. The system includes a mobile app that will facilitate ease of use by crew and medical professionals during training and flight missions.
CHALLENGES FACING APPLICATION OF BIOPHARMACEUTICALS TO PREVENT AND TREAT MEDICAL CONDITIONS IN ASTRONAUTS
NASA has identified more than 400 medical conditions that could require emergency attention during long-term spaceflight missions. Many of these conditions require the use of medication for treatment. It is of utmost importance to ensure that pharmaceutical agents assigned to treat certain medical conditions are efficient during prolonged spaceflight when stored for long periods of time in space. The spaceflight environmental factors which may affect the pharmaceutical properties and integrity of medications include different atmospheric composition, pressures, vibrational forces, ionizing radiation, and prolonged exposure to zero gravity.
Thus far, studies on the effects of these spaceflight environmental conditions on space medications have been made only on conventional pharmaceuticals. At the same time, therapeutic biologic products are at the forefront of medical research and provide the most effective treatments for a variety of spaceflight-related medical conditions such as immune dysfunction, bone desorption, muscle atrophy, radiation sickness, cardiovascular problems, and life-threatening emergency situations. Therefore, application of biopharmaceuticals to treat medical conditions in astronauts seems to be a logical extension to the list of medications for future Mars missions. Because biopharmaceutical drugs have never been used in NASA’s space missions, their efficacy, quality, and pharmaceutical integrity during long storage under spaceflight conditions have never been evaluated. Considering that these characteristics of biopharmaceuticals are more sensitive to environmental conditions than conventional pharmaceutical drugs, extensive research needs to be done during the coming years to select the most appropriate medical products and ensure that a drug product will maintain its safety, quality, efficacy, and physical and chemical characteristics throughout the shelf life required for long-duration missions. Flight surgeons need to be aware of the important issues surrounding the use of biopharmaceuticals. Greater awareness of the potential advantages or problems with products will enable flight surgeons to make informed prescribing decisions, which are critical for astronaut safety.
INVESTIGATION OF BETA-LACTAM ANTIBIOTICS BY IODOMETRIC ASSAY
Beta-lactam antibiotics, renowned for both their ubiquity and extremely effective treatment against bacterial infections, hold one main flaw: their notable instability. Susceptible to degradation through hydrolysis, beta-lactam antibiotics must be closely monitored to ensure adherence to U.S. Food and Drug Administration (FDA) requirements for chemical integrity.
In this project, an iodometric method (originally based upon visual detection of the end products of hydrolysis) was modified to test for quantitative assessment of the integrity of amoxicillin, a beta-lactam antibiotic drug. UV-visible spectrophotometric methods have proven reliable in correlating the amount of iodine reagent applied to an amoxicillin sample and the sample’s percentage of degradation, as the denatured antibiotic absorbs a steady amount of the reagent, resulting in a noteworthy reduction in absorption spectra. Testing of amoxicillin trihydrate tablets, each expiring at different dates, as well as preloaded amoxicillin samples with known percentage of degradation, resulted in the generation of a quantitative curve for drug integrity. In the future, there exists a hopeful outlook for the design of a user-friendly analytical method for determining the intactness of beta-lactam antibiotics hitting the consumer marketplace.
CHANGES IN HUMAN HAIR INDUCED BY ULTRAVIOLET AND GAMMA IRRADIATION
The effects of ultraviolet (UV)- and 137Cs gamma radiation on the structural and chemical integrity of human hair was studied to determine the feasibility of using human hair as a non-invasive biomarker of radiation exposure to ionized gamma and non-ionized UV radiation. In our laboratory, steady state tryptophan (Trp) fluorescence, and chemical analytical methods have been used to evaluate the molecular integrity of Trp fluorophores and SH-groups in hair proteins and to assess the radiation induced damage quantitatively. It was found that human hair fibers were progressively damaged by exposure to both UV- and ionized gamma radiation. Damage to the hair was evidenced by a decrease in the fluorescence intensity as a result of observed depletion of the amino acid tryptophan as well as significant reduction in a number of free SH-groups in hair proteins. Hair damage was dose-dependent for exposures between 0 and 10.0 Gy and 0-20 J/cm2 of UV-radiation.
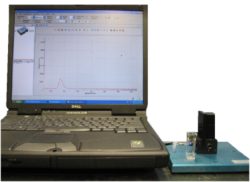
Additional results demonstrate that hair fibers exposed to ionizing radiation undergo smaller changes in Trp fluorescence intensity than when exposed to non-ionizing radiation of similar or lower energy. The stable Trp fluorophore appears to be extremely sensitive to UV radiation in contrast to the ionized gamma radiation whose damage is originated from the reaction of free radicals and direct deposition of energy.
We conclude that fluorescence spectroscopy represents a useful tool in the quantitative evaluation of the radiation exposure and could also be used for the rapid and noninvasive assessment of radiation dose i.e. biodosimeter. The approach is simple, non-invasive and appears to have considerable potential for use as a biological dosimeter utilizing market-available instruments such a portable Ocean Optics Inc. fluorometer, software and fiber optics, which enable quantitative evaluation of radiation dose exposure in a single hair fiber.
EFFECT OF GAMMA IRRADIATION ON THE STRUCTURAL STABILITY AND FUNCTIONAL ACTIVITY OF PLASMA-DERIVED IMMUNOGLOBULINS.
Plasma-originated commercial intravenous immunoglobulin (IgG), which is used for a variety of clinical purposes, has been studied to determine the effect of virus-inactivating doses of gamma irradiation on the structural-functional characteristics of the protein. A detailed analysis has been performed in response to a concern that the use of conventional gamma irradiation may damage biologically active proteins.
The results demonstrate that although gamma irradiation may have some impact on IgG protein structure, the damage can be reduced or even prevented using appropriate irradiation conditions. At the virucidal dose of gamma irradiation (50 kGy) and a temperature of minus 80 degrees C, the integrity of the polypeptide chain of immunoglobulin and the secondary structure of IgG can be completely protected, while conformational changes in tertiary structure are significantly minimized to a level that preserves functional activity. The irradiated IgG retains specific antigen-binding properties and Fc-binding activity, indicating that the conformational integrity of the most important structural regions is not affected by irradiation. These results present strong evidence that gamma irradiation treatment can be effectively implemented for inactivation of pathogens in IgG solutions that are used for intravenous injection.
BENEFICIAL EFFECTS OF METABOLIC SUPPRESSION FOR ADAPTATION AND SURVIVAL IN THE SPACE ENVIRONMENT
In its plans to send humans to deep space destination such as Mars, NASA is facing the risks to health and physiological performance of astronauts caused by exposure to microgravity and space radiation. While most of the environmental conditions inside the spacecraft can closely mimic terrestrial conditions, microgravity cannot yet be managed.
Most spaceflight and ground-based studies suggest that exposure to microgravity leads to significant skeletal muscle atrophy, bone loss, and suppression of total metabolism. Due to the mechanical loading of the body that occurs in microgravity, space station crew members lose up to 1.5% of their skeletal mass and 1.8% of bone strength for each month of mission duration.
Remarkably, certain animals on Earth, including human-sized bears which are largely inactive during 6 to 8 months of hibernation, show no loss in bone mass and less muscle atrophy than would be anticipated over such a prolonged period of physical inactivity. This suggests that animals in a suppressed metabolic state have unique natural mechanisms to prevent disuse muscle and bone atrophy. The molecular mechanisms underlying these important adaptations are not known.
Radiation exposure during spaceflight is the second health-threatening factor that can cause radiation sickness, cancer or death. This study provides new evidence that metabolic activity levels play a critical role in radioprotection. Metabolic suppression, as an adaptive response of cells to minimize damage caused by radiation, enables cells to reduce cellular dysfunction and damage and to prolong their survival despite persistent oxidative stress. Thus mechanistic understanding of metabolism offers a means for sustaining astronauts in long-duration missions.
The ultimate goals of this study are to demonstrate that metabolic suppression induced in animals and humans will profoundly reduce their sensitivity to the damaging effects of radiation and microgravity as well as other kinds of stresses caused by exposure to the space environment. The demonstration of the beneficial effects of suppressed metabolism–induced by different factors such as temperature, nutrition and medications–will have significant effects on mitigation of the most detrimental space environmental factors. Additionally, implementation of a metabolic suppression strategy would radically reduce mission life support requirements.
PHYSIOLOGICAL RESISTANCE TO SPACE RADIATION IN A STATE OF SUSPENDED ANIMATION
The overall objective of this project is to demonstrate that the physiological state under extreme slowing of metabolism (without termination of life processes) provides an effective means to protect against radiation-induced damage.
We are seeking to develop a methodology for reversible induction of a suspended animation-like state in rodents to provide a foundation for reversible induction of this state in humans. The focus will be to investigate radioprotective responses of organisms to biological damage from ionizing radiation. The biological effects of ionizing radiation will be studied by a broad range of quantifiable molecular, genetic, and cellular endpoints up to the integrated tissue level.
This study directly addresses NASA’s interests in examining potential countermeasures capable of protecting astronauts from prolonged exposure to space radiation. Our research objectives are to characterize the hypometabolic state in rodents and evaluate the efficacy of the hypometabolic state in protection against radiation.
NEUROVASCULAR EFFECTS OF HIGH ENERGY PARTICULATE RADIATION: POTENTIAL MOLECULAR TARGETS AND CHEMOPREVENTION
Collaborative project with the Pediatric Infectious Diseases Research Laboratories at the Johns Hopkins University (Dr. D. Grab)
The existing data obtained from in-flight experiments and clinical radiation therapy point to the importance of vascular damage in Central Nervous System (CNS) radiation injury. A consistent and prominent feature of vascular damage after irradiation is disruption of the blood brain barrier (BBB) and blood-spinal cord barrier in both acute and late radiation toxicity. Understanding the molecular networks that are affected in BBB by Low Dose Radiation (LDR) exposure holds the key to uncovering the mechanisms by which radiation deleteriously affects the CNS and how to mitigate these effects.
The overall goals of this work are to provide mechanistic insights into biological effects of high-energy particulate radiation on CNS that account for radiation risks during long-term space missions and to identify potential strategies to mitigate radiation-induce damage. The pathway to reach these goals is to investigate the integrity of BBB during radiation exposure, to identify key factors that are involved in harmful effects of radiation and to assess protective mechanisms against them. This issue is critical for NASA’s human exploration mission plans due to the anticipated extended length of exposure and unpredictable variation of intensity of the space radiation.
PROTECTIVE EFFICACY OF SPECIFIC HUMAN TISSUES UNDER VARIOUS RADIATION EXPOSURE CONDITIONS
The existing data suggest that ionizing radiation of the space environment may induce significant damage to genomic DNA and to collagenized tissues. Potential vascular damage from the radiation may include significant changes in vascular architecture, vessel diameter, and changes in the blood-brain barrier. Chronic exposure to alpha particles causes vascular damage in compact bone resulting in bone infarcts. Long-term radiation induced changes in the blood-brain barrier and vascular changes after exposure to 15-30 Gy particle radiation are noted in the nervous system (recorded by MRI, PET, and CT imaging). These results are consistent with the strong radiation oncology data demonstrating that radiation-induced damage in collagenized tissue including skin, bones, vessels, and connective tissues remains the most morbid complication of radiotherapy. Structural integrity of the perivascular connective tissue, particularly collagen, may play an essential role in these changes.
The aim of the project is to determine the efficacy of several pyruvate-based compounds which might be effective in protecting biological macromolecules, soft tissues and hard tissues receiving high doses of radiation. Pyruvate is natural metabolite of the body that plays a crucial role in the conversion of food to energy. It is an efficient antioxidant and free radical scavenger, and numerous studies have shown that treatment with this compound can be salutary in numerous pathologic conditions. It was recently discovered that mammalian cells secrete pyruvate as an antioxidant defense to oxygen radicals. Since pyruvate is a natural constituent of cells in tissues and has been proven to act as an antioxidant, we are seeking to understand if it can protect the collagenized tissue against radiation-induced damage
DEVELOPMENT OF A NOVEL RADIOPROTECTIVE PHARMACEUTICAL BASED ON MODIFIED STRUCTURE-FUNCTIONAL PROPERTIES OF XI2 PROTEIN ENCODING T-CELL RECEPTOR SUPERANTIGEN
This project is designed to leverage biomedical research on radiation countermeasure technology that are focused on the concrete deliverables – to explore, develop and recommend a novel proprietary biopharmaceutical agent, named XI2 as an agent providing protection of the organism against major space radiation risks.
Studies conducted by NASA Ames Research Center under the Radiation Biology program and Penn State University College of Medicine (lab of Dr. G. Makhatadze) have identified and developed critical enabling technologies and revolutionary scientific potentials designed to serve NASA’s biomedical needs related to long-duration space missions. Using novel biological markers and drug discovery methodologies we are studying the radioprotective potency of XI2 as well as its ability to stimulate immune responses, thereby reducing health risks to astronauts from ionizing radiation exposures. Our approach uses a modified Pseudomonas fluorescens protein encoding T-cell receptor superantigen and assesses its ability to work as a radioprotectant.