Name: Jennifer Eigenbrode
Formal Job Classification: Research astrobiologist
Organization: Code 699, Planetary Environments Laboratory, Space Sciences Directorate
What do you do and what is most interesting about your role here at Goddard? How do you help support Goddard’s mission?
My job is to explore the “organic biogeochemistry” of other bodies in our solar system, such as Mars or ice-covered ocean worlds — like Saturn’s moon Enceladus and Jupiter’s moon Europa. To put it more simply, I look at organic molecules in rocks, ice and sediments and try to figure out where they came from and what happened to them over time. I want to know their story. Are they from life? Were they formed by geological processes in rocks? Are they from meteorites or comets?
As for the big picture, I am fascinated by how life can profoundly change a planet, like it has on Earth. Are there other biospheres? Is there life beyond Earth? If not, why not? I’m intrigued by the prospect that life is unique to Earth and that our home is very special.
I support Goddard’s mission by helping to figure out how to search for life in situ. This involves conducting field and lab studies on samples analogous to planets and moons in our solar system, developing instruments for missions, and figuring out how to use them to answer questions about the prospects of life, within the resources available to a spacecraft.
Why are we looking at organic molecules to search for life?
Organic molecules are the food and the substance of life. Organic molecules made by life have unique characteristics that reflect their origin. Looking at the different types of organic molecules, we may be able to tell how they were made and if they are unique to life.
We don’t know if life beyond Earth will be familiar to us — if it will look like Earth life — however, there are some universal traits of life that get captured in molecules. We seek these signatures. By casting a broad net in terms of the kinds of molecules that we can observe, we increase our chances of discovering them.
How did you get started in astrobiology?
I was a professional student for many years. My career is grounded in geology with a degree from James Madison University. I then started adding in other disciplines, includingbiology and chemistry, with a master’s in geosciences from Indiana University. It wasn’t until I went to Penn State University for my Ph.D. that I discovered Earth system science and became an interdisciplinary astrobiologist. I was part of the first wave of NASA Astrobiology Institute-supported students to graduate with a Ph.D.
For my master’s, I studied Soda Lake in California along the San Andreas Fault. I looked at organic molecules and salt minerals in lake sediment cores to try to understand the history of climate change, such as the Little Ice Age 300 years ago, as opposed to seismic events. Soda Lake is a salt lake, which may be similar to the ancient lakes on Mars. At the time, I had no idea I would be studying Mars in the future.
My experiences at Indiana University got me interested in biogeochemistry and climate evolution. When I got to Penn State, I started connecting the dots: ocean, rocks, atmosphere and life. They all work together as a system. I began wondering: How did our planet evolve all the life that we see today?
Oxygen in the ocean and atmosphere seemed to be at the crux. I packed my bags for months of fieldwork in the Australian outback. There, in the Pilbara region, a rare set of rocks from 2.7 billion years ago preserve a record of ancient lakes and seas. I collected the rocks, shipped them back to the lab at Penn State and started extracting their organic molecules.
The ancient organic molecules told their story. Microbial life used to rely heavily on methane for food and energy and then life transitioned to using oxygen. This transition occurred hundreds of millions of years before Earth’s atmosphere had oxygen. The organisms started making oxygen, triggering reactions with chemicals in rocks and oceans. Life adapted to the changes in their environment. Planet Earth evolved.
The tiniest life on Earth changed the planet forever.
How did you become a science pioneer?
I think I may have been born a science pioneer. I was one of those kids who never stopped asking why. I questioned everything. Surely, I must have driven my parents and some teachers a bit nuts at times, but they encouraged my curiosity and creativity. When I went to college, I discovered science was fun. I knew I was on the right career track because it matched my passion.
What are your current research focuses?
I am fortunate to work with teams of scientists and engineers that together focus on answering big questions about the possibility of extraterrestrial life in the universe. Close to home, Mars and the ocean worlds of our solar system offer the best opportunities to explore this possibility.
As a scientist on the Mars Science Laboratory mission, I explore the rocks in Gale Crater through the imagers and instruments of the Curiosity rover. The rocks tell the story of a 3.6 billion year old lake. I specifically work with the Science Analysis of Mars (SAM) instrument on the rover to look for organic molecules on Mars. We did find some organic molecules, but we are not yet sure how they formed. Furthering our understanding of martian organics is the next step in understanding if life has ever existed on Mars.
I also study how radiation changes organic molecules. Radiation causes chemicals to react. This can happen in martian rocks or in the icy crust of an ocean world. Ultimately, it can obscure the life signatures we are looking for and complicate the search. The more we learn about radiation’s effects, the better we can tailor our search.
A third line of my research is motivated by the possibility that life may exist in the subsurface oceans of Enceladus and Europa. Glacial ice serves as an analog for the icy crust of these ocean worlds, so I led a study on what I thought was the best-case scenario on Earth: the Arctic. We discovered that biosignatures are present in glacial ice, but they are tough to find because the ice dilutes them. This will be one of several challenges to overcome on future missions to ocean worlds, if life exists there.
Why do you do field work in extreme environments?
Astrobiologists go to the field to witness life in action within extreme environments. There are no laboratory substitutes for what can be learned from natural ecosystems in the field. However, there are no field analogs for some extreme environments on other planets and moons in the solar system. For example, Earth is protected from intense ionizing radiation by its magnetic field, so we must study the extreme radiation conditions of other bodies in the lab. In some cases, all we have to work with are laboratory experiments and model simulations. For astrobiology, hypothesis-driven science makes the greatest advances by merging field, laboratory and modeling together. Such advances from Earth-based studies help us design missions to search for life beyond Earth.
Microbial life adapts to cold extreme environments by finding niches that provide the chemical and physical comforts it needs to persists, such as in the pore spaces of rocks or cracks in glacial ice. The environment that matters to organisms can be microscopic or as big as an ocean.
What does it feel like conducting research in the glacial, extreme environments of the Arctic and Antarctic?
The glacial environments of the Arctic and Antarctic are some of the rare, true wildernesses remaining of our world. There is essentially no civilization except what one brings to the field. Near the poles, summer lasts about six weeks, with 24 hours of daylight. The weather is often unpredictable, extreme, and it changes suddenly. There is always wind. Both the Arctic and Antarctic have lots of marine life, including an occasional polar bear in the Arctic. However, in traversing from coast to ice cap everything changes. Both the Arctic and Antarctic still have ice caps. These are great expanses of ice that can cover continents. Standing on an ice cap in Antarctica was an incredibly humbling experience. In every direction, there was nothing but ice and sky as far as the eye could see.
In what other extreme environments have you done field work?
I have done fieldwork in the driest part of the Atacama Desert in the Andes Mountains of Chile. The Atacama Desert is one of the driest places on Earth. It is so dry life that microorganisms have a hard time surviving here. Life depends on water and there is not much, if any, around. As a result, it is very difficult to detect any signs of life here. The Atacama Desert is the closest environment we have for present day Mars. If we want to detect life on Mars, then the Atacama offers the training ground to learn how to do it!
Other field studies that I have been involved in include fieldwork exploring the habitability of lava flows in Hawaii led by Goddard scientist Jacob Bleacher, and searching for life signatures iron-rich springs in Maryland with Marilyn Fogel.
Has your work been featured in documentaries?
I have participated in a few documentaries produced by the Discovery Channel and National Geographic including “Mars Mega Rovers.”
What is the most satisfying thing you have done at Goddard?
Inspiring other people to be curious about the world around them! There is nothing more gratifying than passing on the torch of inspiration to others to carry forward.
Is there something surprising about your hobbies that people do not generally know?
I am a white-water kayaker. My playground is the Potomac River near Great Falls.
By Elizabeth M. Jarrell
NASA’s Goddard Space Flight Center, Greenbelt, Md.
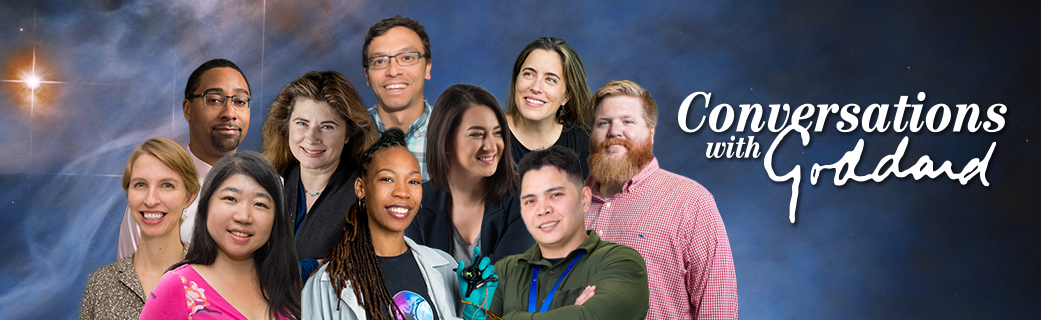
Conversations With Goddard is a collection of Q&A profiles highlighting the breadth and depth of NASA’s Goddard Space Flight Center’s talented and diverse workforce. The Conversations have been published twice a month on average since May 2011. Read past editions on Goddard’s “Our People” webpage.